Սառեցված-հալեցված ցիկլերի վերանայում՝ օգնության հղիության մեջ
Ռամի Վակիմ
«Avenues Life» վերարտադրողական առողջության կենտրոն
Մանչեսթերի քաղաքային համալսարան, Մանչեսթեր, Միացյալ Թագավորություն
Ամփոփագիր
Սառեցված սաղմերի տեղափոխման (FER) ցիկլերն ունեն մի շարք առավելություններ, ներառյալ ձվարանների գերխթանման համախտանիշի (OHSS) ռիսկի նվազեցումը և մեկ սաղմի տեղափոխման խթանումը, ինչն օգնում է նվազեցնել բազմապտուղ հղիությունների քանակը, որոնք կապված են արտամարմնային բեղմնավորման (IVF) հետ։ Կրիոպահպանման բարելավված եղանակները FER-ի հղիության արդյունքները համեմատելի են դարձել թարմ սաղմի տեղափոխման հետ, ինչը հնարավորություն է տալիս առավելագույնի հասցնել ընդհանուր կենդանի ծնունդների թիվը մեկ IVF ցիկլում։ Կարևոր է, որ բեղմնավորման խնամքի մեջ ներգրավված առողջապահական մասնագետները տեղյակ լինեն FER-ի գործելակարգերից և հնարավոր բարդություններից:
DOI 10.54235/27382737-2024.v4.2-36
Introduction
In the early days of in vitro fertilisation (IVF), embryos were created through fertilisation of one or two eggs collected in a natural cycle and pregnancy rates were low [1]. With the introduction of ovarian stimulation, the number of embryos available to transfer in each fresh cycle increased. Consequently, the transfer of multiple embryos was common and those that were not transferred were discarded. In 1983, Trounsen and Mohr [2] described the first pregnancy from a cryopreserved embryo, with the first baby born after frozen–thawed embryo replacement (FER) also known as frozen-thawed embryo transfer (FET) the following year [3].
For many years FER/FET was associated with much lower embryo survival and live-birth rates than fresh IVF. However, recent advances in embryology and a better understanding of how to prepare the endometrium for FET have led to improved outcomes [4, 5]. Data from the Human Fertilisation and Embryology Authority [6] suggest the UK live-birth rate for FER is now similar to that of fresh IVF (Figure 1).
The improvement in outcomes associated with FER has been mirrored by a dramatic increase in the number of FER cycles. Between 2012 and 2017, the number of FER cycles undertaken in the UK rose from 11,959 to 23,828 – an increase of 99% – while the total number of fresh cycles declined by 5%. Frozen cycles now account for more than 34% of all cycles [6]. This review highlights the importance of FER in assisted reproduction; outline the methods of, and indications for, embryo cryopreservation; and discuss the various methods of preparing the endometrium for FER.
Reasons for the increase in frozen–thawed embryo replacement
Improved embryology techniques
The aim of embryo cryopreservation is to preserve the embryo in a state of suspended animation [7]. However, freezing results in the formation of ice crystals which can damage the embryo [8]. This situation is ameliorated through the use of cryoprotectants, which dehydrate the embryo and prevent ice formation.
Initial embryo cryopreservation strategies focused on slow freezing. This involves dehydration of the embryo, achieved by passing it through a series of low concentration cryoprotectants, followed by a controlled freezing process, which takes around 2 hours and requires a cryo machine. Many IVF units now use a freezing technique known as vitrification which is faster and more convenient, taking only minutes and not requiring large, expensive machinery. During vitrification, the embryo is exposed to high concentrations of cryoprotectants and cooled rapidly by exposing it directly or indirectly to liquid nitrogen. The rapidity of the cooling process prevents water from forming ice crystals, effectively solidifying the cells into a glass-like state and avoiding cellular damage [9].
Several studies have highlighted higher embryo survival rates following vitrification compared with slow freezing [4, 10]. This translates into improved clinical outcomes, with a 2015 randomised controlled trial (RCT) [11] demonstrating a higher live-birth rate per embryo thawed after vitrification compared with slow freezing. Moreover, a cohort study of more than 30,000 FERs [12] demonstrated a higher live-birth rate per cycle started for vitrified versus slow frozen embryos, with meta-analysis data [13] supporting these findings. The simplicity, speed and high embryo survival rate (more than 95% post-thaw survival) offered by vitrification have led to its widespread uptake into clinical practice.
Embryos can be cryopreserved at any stage of development from the pronuclear (day 1) and cleavage (days 2–4) stages to blastocyst (days 5 and 6) [14]. During culture, embryos are inspected at different timepoints, with the number of viable embryos falling over time, as some stop developing. This ‘embryonic arrest’ may be due to chromosomal abnormalities, oxidative stress or inability to activate specific genes. Postponing cryopreservation until blastocyst allows embryos to be observed for longer, to better determine which are viable and avoid freezing embryos unnecessarily. Several studies have demonstrated improved live-birth rates following transfer of embryos cryopreserved at the blastocyst stage compared with embryos at earlier stages [7, 15, 16]. It is clear that blastocyst is the preferred stage of embryo freezing in the UK: 98% of the responding clinics (77% of UK clinics responded) favouring cryopreservation at this stage [17].
Widening indications for embryo freezing
Many couples who undergo a fresh IVF cycle will have more than one viable embryo available at the blastocyst stage. Improved outcomes after embryo cryopreservation and FER have allowed clinics to move to a policy of elective single embryo transfer (SET), while maintaining cumulative live-birth rates [18, 19]. Recent years have seen a reduction in the multiple pregnancy rate associated with IVF (Figure 1), with FER playing a central role in this trend [6].
With improved success of embryo cryopreservation, the indications for embryo freezing have widened. Slow-developing embryos, which become blastocysts on day 6, are associated with lower pregnancy rates in fresh cycles than are day-5 blastocysts [20, 21]. This may be partly due to the day of transfer being out of synchrony with the endometrial window of implantation [20, 21]. Several studies demonstrate higher pregnancy rates when day-6 embryos are cryopreserved and resynchronised with the endometrium in a subsequent FER cycle compared with fresh transfer on day 6 [22, 23].
A fresh cycle in which all suitable embryos are frozen is known as a ‘freeze-all’ or ‘freeze-only’ cycle. Below are the indications for a freeze-all strategy. Planned freeze-all permits the use of pre-implantation genetic testing, whereby embryos are biopsied and cryopreserved while genetic analysis is undertaken. It also allows for embryo batching when patients are late in their fertile lives and want more than one child, or for fertility preservation in those due to undergo gonadotoxic therapy. It is yet to be proven, but there may also be benefit to the freeze-all approach in cases of recurrent implantation failure [24, 25].
Indications for freezing all suitable embryos (freeze-all strategy)
Unplanned freeze-all
Ovarian hyperstimulation syndrome
Uterine abnormality identified during ovarian stimulation (e.g. endometrial polyp identified during the cycle, fluid in the endometrium)
Complications of egg-collection procedure (e.g. intraperitoneal bleeding, damage to viscera, pelvic infection)
Social factors (unable to attend embryo transfer or need to defer pregnancy)
Raised progesterone on day of trigger injection (continuing research)
Planned freeze-all
Pre-implantation genetic testing
Fertility preservation
Recurrent implantation failure (continuing research)
A common reason for an unplanned freeze-all strategy is ovarian hyperstimulation syndrome (OHSS). If an embryo implants during a cycle complicated by or at high risk of OHSS, the resultant rise in human chorionic gonadotrophin (hCG) is associated with an increase in the inflammatory mediator vascular endothelial growth factor and a prolonged, more severe clinical course [26]. The freeze-all approach prevents a rise in hCG, avoiding the development of late OHSS. A Cochrane meta-analysis [27] suggests that if the rate of OHSS is 7% following fresh transfer, in the freeze-all approach it is 1–3%. This is clinically significant given the morbidity associated with the condition. Other acute indications for freezing all suitable embryos include pelvic infection, uterine abnormalities (such as fluid in the endometrium) and social factors. The option of freezing all suitable embryos with no adverse effect on cumulative live-birth rate has undoubtedly led to increased flexibility and more individualised patient care in IVF.
Improved outcomes with a planned freeze-all strategy?
Some clinicians advocate a freeze-all strategy in all fresh cycles in an approach known as ‘segmented IVF’ [28]. It is postulated that the supraphysiological hormone levels associated with controlled ovarian stimulation may adversely affect endometrial receptivity, reducing the pregnancy rate and impacting perinatal outcomes [29]. Indeed, the histological changes in the endometrium necessary for implantation occur earlier in controlled ovarian stimulation than in the natural cycle [30-32]. Moreover, controlled ovarian stimulation may negatively affect the expression of proteins essential for implantation, such as integrins [28]. In theory this could be corrected by embryo cryopreservation and subsequent FER. However, this remains controversial.
Pregnancy and live-birth rate
Whether the freeze-all strategy improves clinical pregnancy and live-birth rate compared with fresh embryo transfer in all patients is debatable. A systematic review and meta-analysis in 2013 [33] included three RCTs and demonstrated a higher ongoing pregnancy rate associated with the freeze-all strategy compared with fresh transfer. However, one of the included studies was later retracted due to poor methodology, and a subsequent Cochrane meta-analysis [27] found no difference in live-birth rate between patients undergoing elective freeze-all versus those undergoing fresh transfer. Moreover, two large RCTs published in 2018 [5, 34] demonstrated no difference in live-birth rate between the freeze-all approach and fresh transfer in ovulatory women. These trials excluded patients with polycystic ovarian syndrome (PCOS), who are a group considered to be high responders (i.e. those responding strongly to ovarian stimulation).
In high responders, such as those with high antral follicle counts and PCOS, the case for the freeze-all strategy appears stronger. In 2011 an RCT [35] demonstrated a significantly higher ongoing pregnancy rate with the freeze-all approach compared with fresh transfer in those with a total antral follicle count of more than 15. This finding was supported by a 2016 RCT of 1508 women with PCOS [36], which highlighted a higher live-birth rate with the freeze-all strategy compared with fresh transfer (relative risk [RR] 1.17, 95% confidence interval [CI] 1.05–1.31). Moreover, a 2018 analysis of more than 80,000 cycles from the Society for Assisted Reproduction Technology database in the USA [37] compared the outcomes of women undergoing their first FER after freeze-all approach in their first fresh cycle with those undergoing their first fresh embryo transfer and demonstrated a higher live-birth rate in high responders (15 or more oocytes; 52.0% versus 48.9%, P < 0.02) and a lower live-birth rate in intermediate (6–14 oocytes; 35.3% versus 41.2%, P < 0.02) and low responders (1–5 oocytes; 11.5% versus 25.9%, P < 0.02) associated with the freeze-all strategy. However, caution is warranted, as the reason for the freeze-all approach was poorly documented.
An RCT published in 2019 [38] comparing the freeze-all strategy with fresh embryo transfer included 1650 women and highlighted a significantly higher live-birth rate among those in whom the freeze-all strategy was employed compared with fresh transfer (50% versus 40%; RR 1.26, 95% CI 1.14–1.41). This study excluded those at risk of OHSS; therefore, one would expect the number of high responders to be low. However, closer analysis of the study reveals that the patient cohort was young (mean age 28 years) with a relatively high ovarian response. Moreover, the cumulative live-birth rate associated with embryos derived from the fresh cycle was no different in the freeze-all and fresh transfer groups, and the duration to pregnancy was longer in the freeze-all group. These trends of no difference in cumulative live-birth rate between the freeze-all strategy and fresh transfer, and a longer time to pregnancy associated with the freeze-all approach are consistent across many of the published RCTs [5, 34, 36].
There is some evidence that the freeze-all strategy may be beneficial if there is a premature rise in serum progesterone level in a fresh IVF cycle [39-41]. In fresh IVF a ‘trigger’ injection of hCG or gonadotrophin-releasing hormone (GnRH) agonist is administered around 36 hours before oocyte collection to induce oocyte maturation (mimicking the mid-cycle surge of luteinising hormone [LH] seen in a natural cycle). It is postulated that if the serum progesterone is prematurely elevated on the day of trigger, this may shift the endometrial window of implantation, causing asynchrony between the endometrium and the embryo, negatively impacting implantation. Various thresholds of serum progesterone are suggested of between 2.9 and 6.4 nmol/ml [41-43]. However, more data are required before the freeze-all approach can be widely recommended in this situation.
Maternal and perinatal outcomes
A 2018 meta-analysis [44], which included 26 studies, broadly compared maternal and perinatal outcomes in fresh versus frozen embryo transfer and concluded that, compared with fresh embryo transfer, conception through FER conveyed a lower relative risk of preterm birth (birth before 37 weeks: RR 0.9, 95% CI 0.84–0.97), low birthweight (<2500 g: RR 0.72, 95% CI 0.67–0.77) and small for gestational age (RR 0.61, 95% CI 0.56–0.67). A second systematic review [45] supports these findings. The risk of antepartum haemorrhage, congenital anomalies, perinatal mortality and admission to a neonatal unit appeared similar between fresh and frozen embryo transfer [44, 45]. However, both meta-analyses highlighted an increased RR of gestational hypertensive disorders and postpartum haemorrhage associated with FER. Interestingly, the most recent RCT [38] comparing the freeze-all strategy with fresh transfer has also demonstrated a higher rate of pre-eclampsia (by a factor of 3) in the freeze-all group (RR 3.1, 95% CI 1.06–9.30). There is a well-documented association between FER and large-for-gestational-age babies, with Maheshwari and colleagues’ meta-analysis [44] concluding that the RR of high birthweight (>4500 g) is 1.85 (95% CI 1.46–2.33) compared with fresh transfer. Moreover, a 2016 observational study [46] of more than 112,000 singleton pregnancies demonstrated a higher risk of high birthweight (>4000 g) with FER compared with fresh IVF. These findings are clinically significant considering the association of high birthweight with long-term development of the metabolic syndrome and potentially serious obstetric complications, such as shoulder dystocia and birth trauma [47]. Given the difficulty in predicting these complications in obstetric practice, obstetricians should be aware of the association between conception through FER and high birthweight.
The higher birthweight associated with FER is likely multifactorial. The supraphysiological hormone levels seen in fresh IVF may affect endometrial function interfering with placentation and leading to comparatively lighter babies [48]. Differences in expression of genes between fresh and frozen–thawed embryos are also implicated [49]. In addition, molecular studies describe a higher rate of abnormalities of the intracellular spindle structure necessary for cell division in frozen–thawed compared with fresh blastocysts, which may disrupt the normal mechanisms of cellular division [50].
One RCT is the multicentre E-freeze trial, Elective freezing of embryos versus fresh embryo transfer in IVF: a multicentre randomized controlled trial in the UK (E-Freeze). This study, although limited by sample size, provides no evidence to support the adoption of a routine policy of elective freeze in preference to fresh embryo transfer in order to improve IVF effectiveness in obtaining a healthy baby. There was no evidence of a statistically significant difference in outcomes in the elective freeze group compared to the fresh embryo transfer group: healthy baby rate (20.3% (62/307) versus 24.4% (75/309); risk ratio (RR), 95% CI: 0.84, 0.62 to 1.15}; OHSS (3.6% versus 8.1%; RR, 99% CI: 0.44, 0.15 to 1.30); live birth rate (28.3% versus 34.3%; RR, 99% CI 0.83, 0.65 to 1.06); and miscarriage (14.3% versus 12.9%; RR, 99% CI: 1.09, 0.72 to 1.66). Adherence to allocation was poor in the elective freeze group. The elective freeze approach was more costly and was unlikely to be cost-effective in a UK National Health Service context [51].
Process of frozen–thawed embryo replacement
Endometrial preparation for frozen–thawed embryo replacement
The process of embryo thaw and re-expansion takes around 1–2 hours and is described in Figure 3. Before a cryopreserved embryo can be thawed and transferred, the endometrium must be prepared, such that it is at its most receptive. The endometrium can be prepared using four main methods: the natural cycle, the modified natural cycle, hormone replacement (medicated FER) or ovarian stimulation. Despite the recent rise in the number of FERs, the optimum protocol for preparation of the endometrium is unknown. Prospective data and meta-analyses undertaken between 2013 and 2017 indicate little difference in outcome between each method, but data are limited and protocols vary between clinics, making conclusions difficult to draw [52-55]. Box 2 summarises the characteristics of different methods of endometrial preparation.
Characteristics of different methods of endometrial preparation for frozen–thawed embryo replacement
Natural cycle frozen–thawed embryo replacement
Advantages:
No need for medication (avoids adverse effects)
Cheaper than medicated frozen–thawed embryo replacement (FER)
Disadvantages:
Involves intensive ultrasound and endocrine monitoring
Requires ovulation
No control over day of embryo transfer, as fixed according to ovulation (so embryo transfer may be over a weekend)
Cancellation rate around 8–15% of started cycles
Medicated frozen–thawed embryo replacement
Advantages:
Permits embryo transfer in anovulatory women
Allows choice over day of embryo transfer, which is convenient
In the case of ‘thin endometrium’, the dose and route of estrogen can be modified
Woman may feel more in control of events
Relatively low cycle cancellation rate (1–2%)
Disadvantages:
Prolonged course of medication
Medication may have adverse effects
More expensive than natural cycle FER
Ovarian stimulation frozen–thawed embryo replacement
Advantages:
Permits embryo transfer in anovulatory women who do not want standard medicated FER
May involve fewer days of medication than standard medicated FER
Relies on endogenous estradiol, so it may be beneficial in those with absorption issues
Disadvantages:
Requires intensive monitoring
Medication can cause adverse effects
Gonadotrophins are associated with a risk of ovarian hyperstimulation syndrome
Clomifene may have an adverse effect on the endometrium
Gonadotrophins are expensive
Natural cycle frozen–thawed embryo replacement
Natural cycle FER involves ultrasound and monitoring for signs of ovulation, with embryo transfer timed accordingly (Figure 3). Following FER, embryo implantation and development are supported by the endogenous hormones secreted by the corpus luteum. Given that ovulation occurs in natural cycle FER, abstinence from intercourse or barrier contraception is recommended to reduce the risk of multiple pregnancy through natural conception.
Natural cycle FER avoids medication, which is costly and can have adverse effects. However, the rate of cycle cancellation associated with natural cycle FER is relatively high, with estimates of around 8–15% versus 1–2% for medicated FER [54, 56]. A cycle may be cancelled when no dominant follicle is seen, the LH surge is not diagnosed, bleeding occurs or the endometrium is of insufficient thickness.
There is debate regarding the most effective monitoring strategy to detect ovulation. A common approach involves ultrasound monitoring of the ovaries to identify a dominant follicle, followed by blood and/or urine LH testing. Embryo transfer is usually undertaken on day 6 or 7 after LH surge for a blastocyst stage embryo [57]. Consequently, the date of embryo transfer is fixed in time relative to ovulation. This can be inconvenient when managing clinical workflow, particularly if a clinic is closed at weekends. Moreover, identifying the LH surge represents a challenge. There is variability in the profile of the LH surge between cycles, even in the same woman [58, 59]; the rise in urine LH can lag behind blood by a number of hours and urine monitoring kits have a high false-negative rate [60, 61]. Consequently, some clinics also perform regular ultrasound scans to confirm ovulation and test for the rise in serum progesterone that follows luteinisation.
Modified natural cycle frozen–thawed embryo replacement
An exogenous hCG trigger can be used to more accurately define the time of ovulation in the ‘modified natural cycle’. The dominant follicle is tracked until it is 16–20 mm in diameter, after which an hCG injection is administered (Figure 3). Retrospective data comparing hCG triggering with endocrine monitoring in natural cycle FER are conflicting [62-65], and only two small RCTs have made this comparison [66, 67]. The first involved 124 women and was stopped early because of a low pregnancy rate in the hCG group [66]. The second involved 60 women and demonstrated no difference in clinical pregnancy or live-birth rates [67]. Consequently, there remains debate as to which approach is superior.
The benefit of supplementary luteal phase progesterone in natural cycle FER is unclear. One RCT [68] demonstrated a higher live-birth rate in patients administering vaginal progesterone from the evening of embryo transfer. However, one RCT and several retrospective studies [65, 69-71] have demonstrated no benefit to supplementary progesterone in modified natural cycle FER, with a large retrospective analysis undertaken in 2016 showing no benefit in true natural cycle FER [65]. Despite limited evidence of benefit, there does not appear to be a detrimental effect of supplementary progesterone on pregnancy and live-birth rate.
Hormone replacement (medicated) frozen–thawed embryo replacement
The process of medicated FER is outlined in Figure 5. Medicated FER permits embryo transfer in women with infrequent or no ovulation. It involves administration of oral and/or subcutaneous estrogen to induce endometrial proliferation. The endometrium is monitored via ultrasound and once target criteria are achieved (after approximately 2 weeks of estrogen), progesterone is introduced to encourage endometrial decidualisation. Embryo transfer is usually on the fifth or sixth day of progesterone for blastocysts [57, 72]. However, optimal timing may vary between individuals, with research continuing to identify histological or genomic tests to determine the personalised window of implantation [73-78].
There is reasonable evidence that estrogen can be continued for several weeks before the introduction of progesterone in medicated FER with no adverse effect on clinical pregnancy rate [79]. Consequently, medicated FER offers flexibility over timing of embryo transfer, which is convenient for women and when managing clinic workflow.
During medicated FER it is imperative that the endometrium is not exposed to endogenous progesterone, which could shift the window of implantation. In theory, the high dose of exogenous estrogen should prevent ovulation. However, some protocols involve pituitary downregulation through administration of a GnRH agonist, either subcutaneously or intranasally, for around 3 weeks before commencing estrogen. The benefit of this ‘pituitary suppression’ is unknown, with only a small number of RCTs addressing the issue [80-83]. Given that GnRH agonists prolong the medicated FER cycle and are associated with hypoestrogenic adverse effects, an alternative is daily subcutaneous GnRH antagonist injections given alongside estrogen to block pituitary and ovarian activity directly. The use of GnRH antagonist in medicated FER has been insufficiently studied and the results of a continuing RCT comparing medicated FER with and without GnRH antagonist are keenly awaited (clinicaltrials.gov, NCT03763786).
Ovarian stimulation cycles
Frozen–thawed embryos can also be transferred as part of a mild ovarian stimulation cycle. This offers patients with ovulatory problems an alternative to standard hormone replacement FER. Traditional ovulation induction regimens involve low-dose gonadotrophin injections or clomifene. The limited available evidence shows equivalent pregnancy rates between gonadotrophin FER and both medicated and natural cycle FER [84-86]. However, gonadotrophin protocols are complicated, involve intensive monitoring and carry a risk of OHSS. Consequently, the use of gonadotrophin for FER is uncommon. Similarly, clomifene is not widely used in FER, as it may have an anti-estrogen effect on the endometrium and has been associated with a thin endometrium with abnormal subendometrial blood flow [55, 87, 88].
There has been recent interest in the aromatase inhibitor letrozole, which is used as an ovulation induction agent in PCOS. Patients taking letrozole have increased endometrial expression of integrin, a marker of endometrial receptivity, suggesting that letrozole may aid implantation [87-90]. Letrozole FER requires fewer days of medication than medicated FER and small retrospective studies suggest at least equivalent clinical pregnancy rates [91-93].
Endometrial thickness in frozen–thawed embryo replacement
The minimum endometrial thickness needed before FER is controversial, with a cut-off of 7 mm often used on the basis of meta-analysis data from fresh IVF cycles [94]. However, in an analysis of 768 medicated FER cycles [95], a higher pregnancy rate was demonstrated with an endometrial thickness of 9–14 mm compared with 7–8 mm. Others have demonstrated a poor correlation between endometrial thickness and outcome, instead favouring more complex criteria involving endometrial pattern [96]. However, these more complex criteria can be difficult to translate into routine clinical practice.
A 2018 analysis of more than 18 000 FERs from the Canadian ART Registry [97] demonstrated a reduction in clinical pregnancy and live-birth rates with each millimetre decrease in endometrial thickness below 7 mm. The difference in live-birth rate between women with an endometrial thickness of more than 7 mm and those with a thickness of 6.0–6.9 mm was 4.6% (odds ratio 1.29, 95% CI 1.03–1.62). However, it is important to balance the decrease in live-birth rate associated with thin endometrium against the effect that recurrently cancelling cycles may have on the cumulative chance of live birth over the long term. While it is prudent to try different methods of endometrial preparation in those with thin endometrium, in some an endometrial thickness greater than 7 mm will be unachievable.
Calculation of estimated due date
The day of egg collection in fresh IVF is akin to ovulation in the natural cycle, which occurs around 2 weeks after the first day of the last menstrual period. Consequently, the theoretical gestational age of a pregnancy at the time of fresh or frozen embryo transfer is 2 weeks plus the age of the embryo (in other words, 2 weeks and 5 days for a blastocyst). The pregnancy test is normally undertaken 11 days after blastocyst transfer, which is 4 weeks and 2 days of gestation.
Consent for use of cryopreserved embryos
When embryos are owned by two people, having been created using their own or donor gametes, UK law permits the freezing, storage and transfer of these embryos only with the consent of both people (in the case of surrogacy consent of the surrogate would also be required for transfer) [98].
In cases of fertility preservation before fertility-compromising treatment, storing of an individual’s gametes rather than embryos may be preferable to prevent future withdrawal of consent.
Conclusion
The number and proportion of FER cycles undertaken in the UK and worldwide has increased dramatically over recent years. It is important for general obstetricians and gynaecologists to be aware of this trend, as well as the process of FER and the potential issues that may arise both during the cycle and in any resulting pregnancy.
Improved embryo cryopreservation techniques have resulted in outcomes for FER that are similar to fresh embryo transfer. FER maximises the cumulative live-birth rate of a fresh in vitro fertilisation (IVF) cycle by using excess embryos and encourages a policy of single embryo transfer; this has contributed to a fall in the multiple pregnancy rate associated with IVF.Freezing all suitable embryos in a fresh cycle reduces the risk of ovarian hyperstimulation syndrome.
The endometrium is prepared for FER by a natural or medicated protocol; the optimum method is unknown.
References
Steptoe PC, Edwards RG. Birth after the reimplantation of a human embryo. Lancet 1978; 2: 366.
Trounson A, Mohr L. Human pregnancy following cryopreservation, thawing and transfer of an eight-cell embryo. Nature 1983; 305: 707–9.
Zeilmaker GH, Alberda AT, van Gent I, Rijkmans CM, Drogendijk AC. Two pregnancies following transfer of intact frozen-thawed embryos. Fertil Steril 1984; 42: 293–6.
Loutradi KE, Kolibianakis EM, Venetis CA, Papanikolaou EG, Pados G, Bontis I, et al. Cryopreservation of human embryos by vitrification or slow freezing: a systematic review and meta-analysis. Fertil Steril 2008; 90: 186–93.
Vuong LN, Dang VQ, Ho TM, Huynh BG, Ha DT, Pham TD, et al. IVF transfer of fresh or frozen embryos in women without polycystic ovaries. N Engl J Med 2018; 378: 137–47.
Human Fertilisation and Embryology Authority . Fertility Treatment 2017: Trends and Figures. London: HFEA; 2019
Wong KM, Mastenbroek S, Repping S. Cryopreservation of human embryos and its contribution to in vitro fertilization success rates. Fertil Steril 2014; 102: 19–26.
Mazur P. Kinetics of water loss from cells at subzero temperatures and the likelihood of intracellular freezing. J Gen Physiol 1963; 47: 347–69.
Rall WF, Fahy GM. Ice free cryopreservation of mouse embryos at –196 degrees C by vitrification. Nature 1985; 313: 573–5.
Balaban B, Urman B, Ata B, Isiklar A, Larman MG, Hamilton R, et al. A randomized controlled study of human Day 3 embryo cryopreservation by slow freezing or vitrification: vitrification is associated with higher survival, metabolism and blastocyst formation. Hum Reprod 2008; 23: 1976–82.
Debrock S, Peeraer K, Fernandez Gallardo E, De Neubourg D, Spiessens C, D’Hooghe TM. Vitrification of cleavage stage day 3 embryos results in higher live birth rates than conventional slow freezing: a RCT. Hum Reprod 2015; 30: 1820–30.
Li Z, Wang YA, Ledger W, Edgar DH, Sullivan EA. Clinical outcomes following cryopreservation of blastocysts by vitrification or slow freezing: a population-based cohort study. Hum Reprod 2014; 29: 2794–801.
Rienzi L, Gracia C, Maggiulli R, LaBarbera AR, Kaser DJ, Ubaldi FM, et al. Oocyte, embryo and blastocyst cryopreservation in ART: systematic review and meta-analysis comparing slow-freezing versus vitrification to produce evidence for the development of global guidance. Hum Reprod Update 2017; 23: 139–55.
Edgar DH, Gook DA. A critical appraisal of cryopreservation (slow cooling versus vitrification) of human oocytes and embryos. Hum Reprod Update 2012; 18: 536–54.
Holden EC, Kashani BN, Morelli SS, Alderson D, Jindal SK, Ohman-Strickland PA, et al. Improved outcomes after blastocyst-stage frozen-thawed embryo transfers compared with cleavage stage: a Society for Assisted Reproductive Technologies Clinical Outcomes Reporting System study. Fertil Steril 2018; 110: 89–94.e2.
Surrey E, Keller J, Stevens J, Gustofson R, Minjarez D, Schoolcraft W. Freeze-all: enhanced outcomes with cryopreservation at the blastocyst stage versus pronuclear stage using slow-freeze techniques. Reprod Biomed Online 2010; 21: 411–7.
Noble M, Child T. Frozen-thawed embryo transfer (FET): A UK-wide survey of IVF clinics highlighting wide variation in practice and an analysis of 578 patients comparing GnRH-antagonist with GnRH-agonist pituitary suppression in medicated FET. BJOG 2019; 126(S2): 194.
McLernon DJ, Harrild K, Bergh C, Davies MJ, De Neubourg D, Dumoulin JCM, et al. Clinical effectiveness of elective single versus double embryo transfer: meta-analysis of individual patient data from randomised trials. BMJ 2010; 341: c6945.
Pandian Z, Marjoribanks J, Ozturk O, Serour G, Bhattacharya S. Number of embryos for transfer following in vitro fertilisation or intra-cytoplasmic sperm injection. Cochrane Database Syst Rev 2013; 7: CD003416.
Shapiro BS, Richter KS, Harris DC, Daneshmand ST. A comparison of day 5 and day 6 blastocyst transfers. Fertil Steril 2001; 75: 1126–30.
Fragouli E, Alfarawati S, Spath K, Wells D. Morphological and cytogenetic assessment of cleavage and blastocyst stage embryos. Mol Hum Reprod 2014; 20: 117–26.
Shapiro BS, Daneshmand ST, Garner FC, Aguirre M, Ross R. Contrasting patterns in in vitro fertilization pregnancy rates among fresh autologous, fresh oocyte donor and cryopreserved cycles with the use of day 5 or day 6 blastocysts may reflect differences in embryo-endometrium synchrony. Fertil Steril 2008; 89: 20–6.
Richter KS, Shipley SK, McVearry I, Tucker MJ, Widra EA. Cryopreserved embryo transfers suggest that endometrial receptivity may contribute to reduced success rates of later developing embryos. Fertil Steril 2006; 86: 862–6.
Magdi Y, El-Damen A, Fathi AM, Abdelaziz AM, Abd-Elfatah Youssef M, Abd-Allah AA-E, et al. Revisiting the management of recurrent implantation failure through freeze-all policy. Fertil Steril 2017; 108: 72–7.
Shapiro BS, Garner FC. Recurrent implantation failure is another indication for the freeze-all strategy. Fertil Steril 2017; 108: 44.
Humaidan P, Nelson SM, Devroey P, Coddington CC, Schwartz LB, Gordon K, et al. Ovarian hyperstimulation syndrome: review and new classification criteria for reporting in clinical trials. Hum Reprod 2016; 31: 1997–2004.
Wong KM, van Wely M, Mol F, Repping S, Mastenbroek S. Fresh versus frozen embryo transfers in assisted reproduction. Cochrane Database Syst Rev 2017; 3: CD011184.
Weinerman R, Mainigi M. Why we should transfer frozen instead of fresh embryos: the translational rationale. Fertil Steril 2014; 102: 10–8.
Bourgain C, Devroey P. The endometrium in stimulated cycles for IVF. Hum Reprod Update 2003; 9: 515–22.
Lass A, Peat D, Avery S, Brinsden P. Histological evaluation of endometrium on the day of oocyte retrieval after gonadotrophin-releasing hormone agonist-follicle stimulating hormone ovulation induction for in-vitro fertilization. Hum Reprod 1998; 13: 3203–5.
Nikas G, Develioglu OH, Toner JP, Jones HW. Endometrial pinopodes indicate a shift in the window of receptivity in IVF cycles. Hum Reprod 1999; 14: 787–92.
Zapantis G, Szmyga MJ, Rybak EA, Meier UT. Premature formation of nucleolar channel systems indicates advanced endometrial maturation following controlled ovarian hyperstimulation. Hum Reprod 2013; 28: 3292–300.
Roque M, Lattes K, Serra S, Solà I, Geber S, Carreras R, et al. Fresh embryo transfer versus frozen embryo transfer in in vitro fertilization cycles: a systematic review and meta-analysis. Fertil Steril 2013; 99: 156–62.
Shi Y, Sun Y, Hao C, Zhang H, Wei D, Zhang Y, et al. Transfer of fresh versus frozen embryos in ovulatory women. N Engl J Med 2018; 378: 126–36.
Shapiro BS, Daneshmand ST, Garner FC, Aguirre M, Hudson C, Thomas S. Evidence of impaired endometrial receptivity after ovarian stimulation for in vitro fertilization: a prospective randomized trial comparing fresh and frozen-thawed embryo transfers in high responders. Fertil Steril 2011; 96: 516–8.
Chen Z-J, Shi Y, Sun Y, Zhang B, Liang X, Cao Y, et al. Fresh versus frozen embryos for infertility in the polycystic ovary syndrome. N Engl J Med 2016; 375: 523–33.
Acharya KS, Acharya CR, Bishop K, Harris B, Raburn D, Muasher SJ. Freezing of all embryos in in vitro fertilization is beneficial in high responders, but not intermediate and low responders: an analysis of 82,935 cycles from the Society for Assisted Reproductive Technology registry. Fertil Steril 2018; 110: 880–7.
Wei D, Liu JY, Sun Y, Shi Y, Zhang B, Liu JQ, et al. Frozen versus fresh single blastocyst transfer in ovulatory women: a multicentre, randomised controlled trial. Lancet 2019; 393: 1310–8.
Healy MW, Patounakis G, Connell MT, Devine K, DeCherney AH, Levy MJ, et al. Does a frozen embryo transfer ameliorate the effect of elevated progesterone seen in fresh transfer cycles? Fertil Steril 2016; 105: 93–9.e1.
Yang S, Pang T, Li R, Yang R, Zhen X, Chen X, et al. The individualized choice of embryo transfer timing for patients with elevated serum progesterone level on the HCG day in IVF/ICSI cycles: a prospective randomized clinical study. Gynecol Endocrinol 2015; 31: 355–8.
Ochsenkühn R, Arzberger A, von Schönfeldt V, Gallwas J, Rogenhofer N, Crispin A, et al. Subtle progesterone rise on the day of human chorionic gonadotropin administration is associated with lower live birth rates in women undergoing assisted reproductive technology: a retrospective study with 2,555 fresh embryo transfers. Fertil Steril 2012; 98: 347–54.
Bosch E, Labarta E, Crespo J, Simón C, Remohí J, Jenkins J, et al. Circulating progesterone levels and ongoing pregnancy rates in controlled ovarian stimulation cycles for in vitro fertilization: analysis of over 4000 cycles. Hum Reprod 2010; 25: 2092–100.
Shapiro BS, Daneshmand ST, Garner FC, Aguirre M, Hudson C, Thomas S. Embryo cryopreservation rescues cycles with premature luteinization. Fertil Steril 2010; 93: 636–41.
Maheshwari A, Pandey S, Amalraj Raja E, Shetty A, Hamilton M, Bhattacharya S. Is frozen embryo transfer better for mothers and babies? Can cumulative meta-analysis provide a definitive answer? Hum Reprod Update 2018; 24: 35–58.
Sha T, Yin X, Cheng W, Massey IY. Pregnancy-related complications and perinatal outcomes resulting from transfer of cryopreserved versus fresh embryos in vitro fertilization: a meta-analysis. Fertil Steril 2018; 109: 330–9.
Maheshwari A, Raja EA, Bhattacharya S. Obstetric and perinatal outcomes after either fresh or thawed frozen embryo transfer: an analysis of 112,432 singleton pregnancies recorded in the Human Fertilisation and Embryology Authority anonymized dataset. Fertil Steril 2016; 106: 1703–8.
Dyachenko A, Ciampi A, Fahey J, Mighty H, Oppenheimer L, Hamilton EF. Prediction of risk for shoulder dystocia with neonatal injury. Am J Obstet Gynecol 2006; 195: 1544–9.
Evans J, Hannan NJ, Edgell TA, Vollenhoven BJ, Lutjen PJ, Osianlis T, et al. Fresh versus frozen embryo transfer: backing clinical decisions with scientific and clinical evidence. Hum Reprod Update 2014; 20: 808–21.
Shaw L, Sneddon SF, Brison DR, Kimber SJ. Comparison of gene expression in fresh and frozen-thawed human preimplantation embryos. Reproduction 2012; 144: 569–82.
Chatzimeletiou K, Morrison EE, Panagiotidis Y, Vanderzwalmen P, Prapas N, Prapas Y, et al. Cytoskeletal analysis of human blastocysts by confocal laser scanning microscopy following vitrification. Hum Reprod 2011; 27: 106–13.
E-Freeze. Randomized Controlled Trial: Hum Reprod 2022 Mar 1;37(3):476 487.doi10.1093/humrep/deab279.
Groenewoud ER, Cantineau AEP, Kollen BJ, Macklon NS, Cohlen BJ. What is the optimal means of preparing the endometrium in frozen–thawed embryo transfer cycles? A systematic review and meta-analysis. Hum Reprod Update 2013; 19: 458–70.
Groenewoud ER, Cohlen BJ, Al-Oraiby A, Brinkhuis EA, Broekmans FJM, de Bruin JP, et al. A randomized controlled, non-inferiority trial of modified natural versus artificial cycle for cryo-thawed embryo transfer. Hum Reprod 2016; 31: 1483–92.
Mounce G, McVeigh E, Turner K, Child TJ. Randomized, controlled pilot trial of natural versus hormone replacement therapy cycles in frozen embryo replacement in vitro fertilization. Fertil Steril 2015; 104: 915–20.e1.
Ghobara T, Gelbaya TA, Ayeleke RO. Cycle regimens for frozen-thawed embryo transfer. Cochrane Database Syst Rev 2017; 7: CD003414.
Sathanandan M, Macnamee MC, Rainsbury P, Wick K, Brinsden P, Edwards RG. Replacement of frozen-thawed embryos in artificial and natural cycles: a prospective semi-randomized study. Hum Reprod 1991; 6: 685–7.
Mackens S, Santos-Ribeiro S, van de Vijver A, Racca A, Van Landuyt L, Tournaye H, et al. Frozen embryo transfer: a review on the optimal endometrial preparation and timing. Hum Reprod 2017; 32: 2234–42.
Ecochard R, Bouchard T, Leiva R, Abdulla S, Dupuis O, Duterque O, et al. Characterization of hormonal profiles during the luteal phase in regularly menstruating women. Fertil Steril 2017; 108: 175–82.e1.
Park SJ, Goldsmith LT, Skurnick JH, Wojtczuk A, Weiss G. Characteristics of the urinary luteinizing hormone surge in young ovulatory women. Fertil Steril 2007; 88: 684–90.
Miller PB, Soules MR. The usefulness of a urinary LH kit for ovulation prediction during menstrual cycles of normal women. Obstet Gynecol 1996; 87: 13–7.
Ghazeeri GS, Vongprachanh P, Kutteh WH. The predictive value of five different urinary LH kits in detecting the LH surge in regularly menstruating women. Int J Fertil Womens Med 2000; 45: 321–6.
Chang EM, Han JE, Kim YS, Lyu SW, Lee WS, Yoon TK. Use of the natural cycle and vitrification thawed blastocyst transfer results in better in-vitro fertilization outcomes: cycle regimens of vitrification thawed blastocyst transfer. J Assist Reprod Genet 2011; 28: 369–74.
Tomás C, Alsbjerg B, Martikainen H, Humaidan P. Pregnancy loss after frozen-embryo transfer: a comparison of three protocols. Fertil Steril 2012; 98: 1165–9.
Weissman A. What is the preferred method for timing natural cycle frozen-thawed embryo transfer? Reprod Biomed Online 2009; 19: 66–71.
Montagut M, Santos-Ribeiro S, De Vos M, Polyzos NP, Drakopoulos P, Mackens S, et al. Frozen-thawed embryo transfers in natural cycles with spontaneous or induced ovulation: the search for the best protocol continues. Hum Reprod 2016; 31: 2803–10.
Fatemi HM, Kyrou D, Bourgain C, Van den Abbeel E, Griesinger G, Devroey P. Cryopreserved-thawed human embryo transfer: spontaneous natural cycle is superior to human chorionic gonadotropin-induced natural cycle. Fertil Steril 2010; 94: 2054–8.
Weissman A, Horowitz E, Ravhon A, Steinfeld Z, Mutzafi R, Golan A, et al. Spontaneous ovulation versus HCG triggering for timing natural-cycle frozen thawed embryo transfer: a randomized study. Reprod Biomed Online 2011; 23: 484–9.
Bjuresten K, Landgren B-M, Hovatta O, Stavreus-Evers A. Luteal phase progesterone increases live birth rate after frozen embryo transfer. Fertil Steril 2011; 95: 534–7.
Eftekhar M, Rahsepar M, Rahmani E. Effect of progesterone supplementation on natural frozen-thawed embryo transfer cycles: a randomized controlled trial. Int J Fertil Steril 2013; 7: 13–20.
Kim CH, Lee YJ, Lee K-H, Kwon SK, Kim SH, Chae HD, et al. The effect of luteal phase progesterone supplementation on natural frozen-thawed embryo transfer cycles. Obstet Gynecol Sci 2014; 57: 291–6.
Kyrou D, Fatemi HM, Popovic-Todorovic B, Van den Abbeel E, Camus M, Devroey P. Vaginal progesterone supplementation has no effect on ongoing pregnancy rate in HCG-induced natural frozen-thawed embryo transfer cycles. Eur J Obstet Gynecol Reprod Biol 2010; 150: 175–9.
Glujovsky D, Pesce R, Fiszbajn G, Sueldo C, Hart RJ, Ciapponi A. Endometrial Preparation for Women Undergoing Embryo Transfer with Frozen Embryos or Embryos Derived from Donor Oocytes. Chichester: John Wiley & Sons, Ltd; 2010.
Murray MJ, Meyer WR, Zaino RJ, Lessey BA, Novotny DB, Ireland K, et al. A critical analysis of the accuracy, reproducibility and clinical utility of histologic endometrial dating in fertile women. Fertil Steril 2004; 81: 1333–43.
Gomaa H, Casper RF, Esfandiari N, Bentov Y. Non-synchronized endometrium and its correction in non-ovulatory cryopreserved embryo transfer cycles. Reprod Biomed Online 2015; 30: 378–84.
Ruiz-Alonso M, Blesa D, Díaz-Gimeno P, Gómez E, Fernández-Sánchez M, Carranza F, et al. The endometrial receptivity array for diagnosis and personalized embryo transfer as a treatment for patients with repeated implantation failure. Fertil Steril 2013; 100: 818–24.
Díaz-Gimeno P, Horcajadas JA, Martínez-Conejero JA, Esteban FJ, Alamá P, Pellicer A, et al. A genomic diagnostic tool for human endometrial receptivity based on the transcriptomic signature. Fertil Steril 2011; 95: 50–60, 60.e1–15.
Bassil R, Casper R, Samara N, Hsieh T-B, Barzilay E, Orvieto R, et al. Does the endometrial receptivity array really provide personalized embryo transfer? J Assist Reprod Genet 2018; 35: 1301–5.
Shi C, Han HJ, Fan LJ, Guan J, Zheng XB, Chen X, et al. Diverse endometrial mRNA signatures during the window of implantation in patients with repeated implantation failure. Human Fertility (Camb) 2018; 21: 183–94.
Soares SR, Troncoso C, Bosch E, Serra V, Simón C, Remohí J, et al. Age and uterine receptiveness: predicting the outcome of oocyte donation cycles. J Clin Endocrinol Metab 2005; 90: 4399–404.
Dal Prato L, Borini A, Cattoli M, Bonu MA, Sciajno R, Flamigni C. Endometrial preparation for frozen-thawed embryo transfer with or without pretreatment with gonadotropin-releasing hormone agonist. Fertil Steril 2002; 77: 956–60.
Azimi Nekoo E, Chamani M, Shahrokh Tehrani E, Hossein Rashidi B, Davari Tanha F, Kalantari V. Artificial endometrial preparation for frozen-thawed embryo transfer with or without pretreatment with depot gonadotropin releasing hormone agonist in women with regular menses. J Family Reprod Health 2015; 9: 1–4.
Simon A, Hurwitz A, Zentner BS, Bdolah Y, Laufer N. Transfer of frozen-thawed embryos in artificially prepared cycles with and without prior gonadotrophin-releasing hormone agonist suppression: a prospective randomized study. Hum Reprod 1998; 13: 2712–7.
El-Toukhy T. Pituitary suppression in ultrasound-monitored frozen embryo replacement cycles. A randomised study. Hum Reprod 2004; 19: 874–9.
Wright KP, Guibert J, Weitzen S, Davy C, Fauque P, Olivennes F. Artificial versus stimulated cycles for endometrial preparation prior to frozen-thawed embryo transfer. Reprod Biomed Online 2006; 13: 321–5.
Peeraer K, Couck I, Debrock S, De Neubourg D, De Loecker P, Tomassetti C, et al. Frozen-thawed embryo transfer in a natural or mildly hormonally stimulated cycle in women with regular ovulatory cycles: a RCT. Hum Reprod 2015; 30: 2552–62.
Yarali H, Polat M, Mumusoglu S, Yarali I, Bozdag G. Preparation of endometrium for frozen embryo replacement cycles: a systematic review and meta-analysis. J Assist Reprod Genet 2016; 33: 1287–304.
Baruah J, Roy KK, Rahman SM, Kumar S, Sharma JB, Karmakar D. Endometrial effects of letrozole and clomiphene citrate in women with polycystic ovary syndrome using spiral artery Doppler. Arch Gynecol Obstet 2009; 279: 311–4.
Gonen Y, Casper RF. Sonographic determination of a possible adverse effect of clomiphene citrate on endometrial growth. Hum Reprod 1990; 5: 670–4.
Ganesh A, Chauhan N, Das S, Chakravarty B, Chaudhury K. Endometrial receptivity markers in infertile women stimulated with letrozole compared with clomiphene citrate and natural cycles. Syst Biol Reprod Med 2014; 60: 105–11.
Thomas K, Thomson A, Wood S, Kingsland C, Vince G, Lewis-Jones I. Endometrial integrin expression in women undergoing in vitro fertilization and the association with subsequent treatment outcome. Fertil Steril 2003; 80: 502–7.
Hu YJ, Chen YZ, Zhu YM, Huang HF. Letrozole stimulation in endometrial preparation for cryopreserved-thawed embryo transfer in women with polycystic ovarian syndrome: a pilot study. Clin Endocrinol (Oxf) 2014; 80: 283–9.
Li SJ, Zhang YJ, Chai XS, Nie MF, Zhou YY, Chen JL, et al. Letrozole ovulation induction: an effective option in endometrial preparation for frozen-thawed embryo transfer. Arch Gynecol Obstet 2013; 289: 687–93.
Munch EM, Burger NZ. Uterine preparation prior to frozen embryo transfer using letrozole: a case series. Fertil Steril 2018; 109: e39.
Kasius A, Smit JG, Torrance HL, Eijkemans MJC, Mol BW, Opmeer BC, et al. Endometrial thickness and pregnancy rates after IVF: a systematic review and meta-analysis. Hum Reprod Update 2014; 20: 530–41.
El-Toukhy T, Coomarasamy A, Khairy M, Sunkara K, Seed P, Khalaf Y, et al. The relationship between endometrial thickness and outcome of medicated frozen embryo replacement cycles. Fertil Steril 2008; 89: 832–9.
Gingold JA, Lee JA, Rodriguez-Purata J, Whitehouse MC, Sandler B, Grunfeld L, et al. Endometrial pattern, but not endometrial thickness, affects implantation rates in euploid embryo transfers. Fertil Steril 2015; 104: 620–5.
Liu KE, Hartman M, Hartman A, Luo ZC, Mahutte N. The impact of a thin endometrial lining on fresh and frozen-thaw IVF outcomes: an analysis of over 40 000 embryo transfers. Hum Reprod 2018; 33: 1883–8.
HM Government. The Human Fertilisation and Embryology (Statutory Storage Period for Embryos and Gametes) Regulations 2009. London: The Stationery Office; 2009
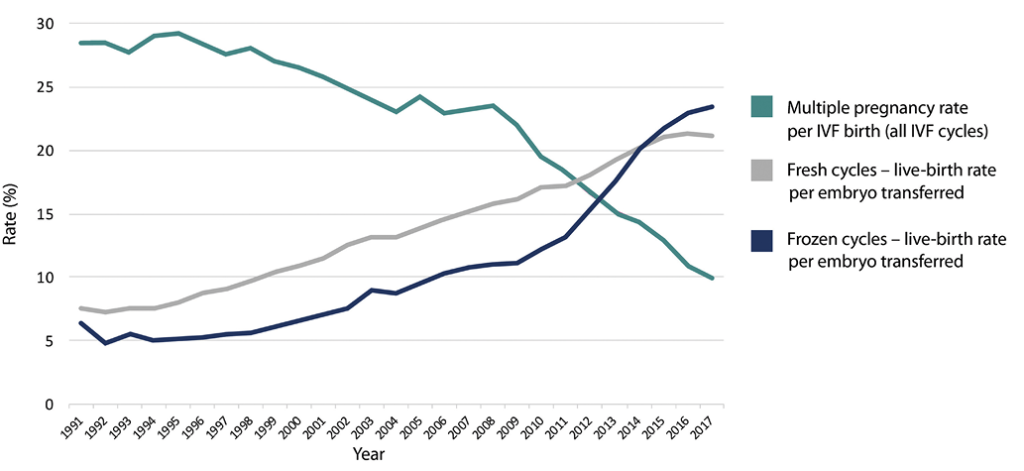
Figure 1. Live-birth rate per embryo transferred and multiple birth rate per live birth (UK). Constructed using Human Fertilisation and Embryology Authority data [6]. IVF = in vitro fertilisation.

Figure 2. Microscopic (x200 magnification) images of blastocyst embryos during thaw (with consent of patients). (A) A collapsed embryo. At the time of embryo cryopreservation, embryos are collapsed by disruption of the TE. This is done either mechanically or by laser. The aim of this is to reduce the water content and avoid ice crystal formation. (B) AH. It is possible that the outer coat of the embryo, the ZP, may become hardened during cryopreservation. To help the embryo hatch, a portion of the ZP may be removed at the time of thaw using a laser. (C) A thawed and re-expanded embryo. Embryos are thawed by passing through a series of decreasing concentration cryoprotectants. Expansion of the embryo occurs over around 1–2 hours. (D) A thawed blastocyst hatching through the ZP. The cross marking in the images is the guide for the laser used for AH. AH = assisted hatching; TE = trophectoderm; ZP = zona pellucida.
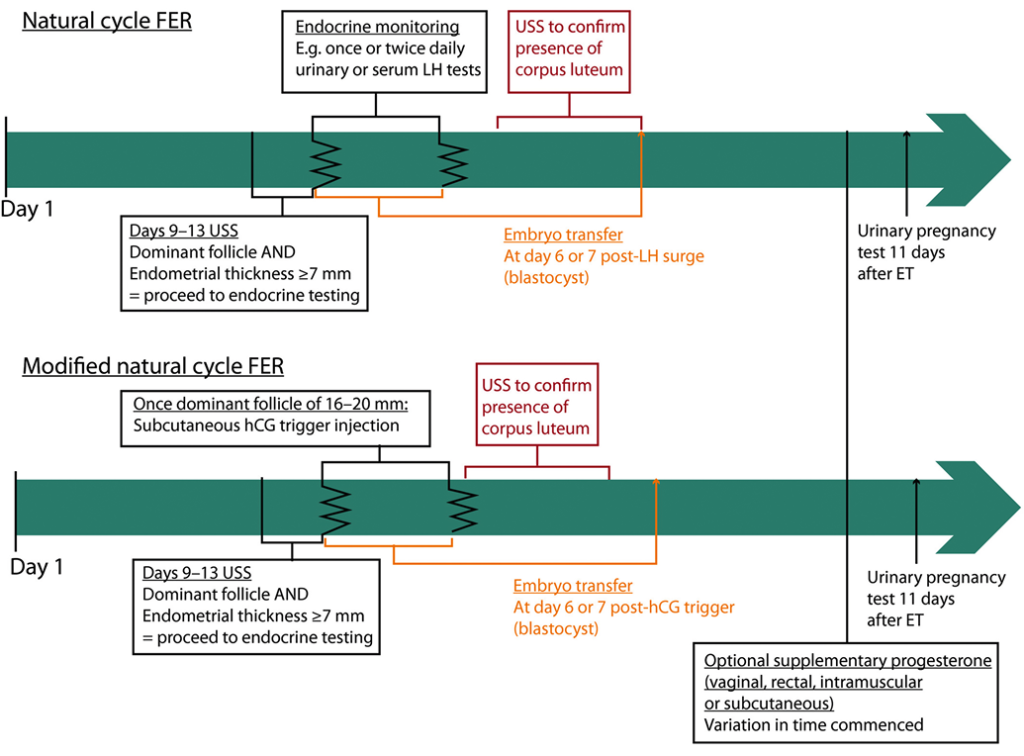
Figure 3: Natural cycle and modified natural cycle FER example cycle timelines. The timeline starts at day 1, which is the first day of the menstrual period. Variation exists between clinics, particularly with regard to the methods used to determine the time of ovulation. ET = embryo transfer; FER = frozen–thawed embryo replacement; hCG = human chorionic gonadotrophin; LH = luteinising hormone; USS = ultrasound scan.
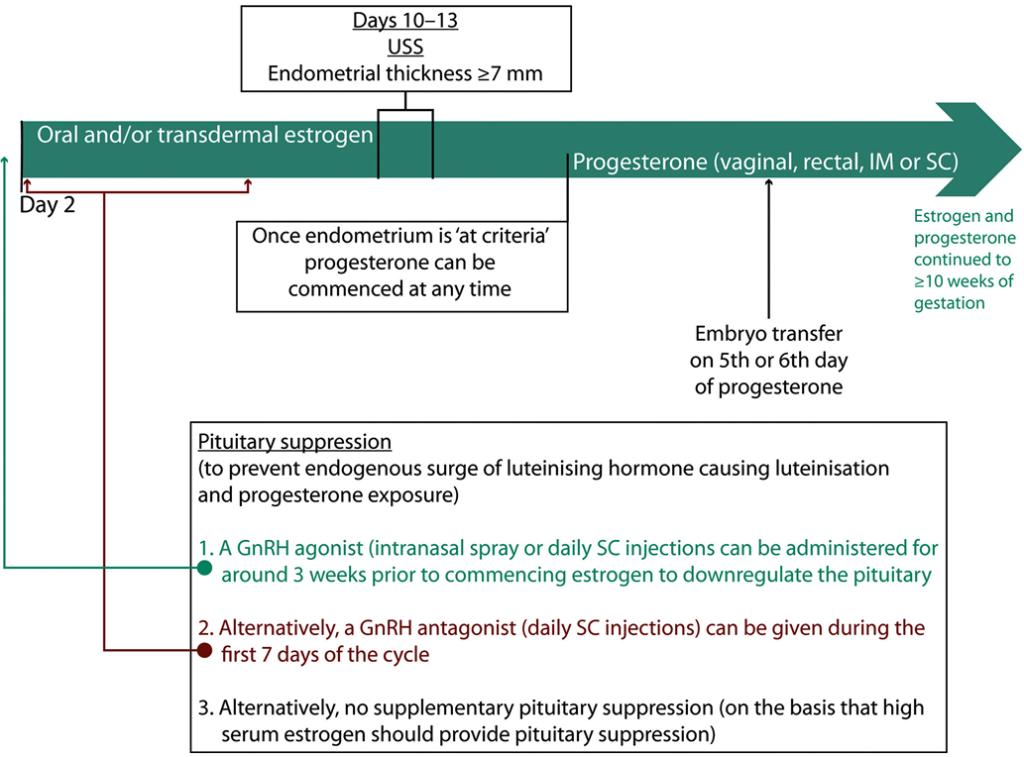
Figure 4: Hormone replacement (medicated) FER example cycle timeline. The cycle starts on day 2 of the menstrual period. Variation exists between clinics, particularly with regard to the use of pituitary suppression, the route of estrogen and progesterone, and the criteria used to assess the endometrium on ultrasound. FER = frozen–thawed embryo replacement; GnRH = gonadotrophin-releasing hormone; IM = intramuscular; SC = subcutaneous; USS = ultrasound scan.